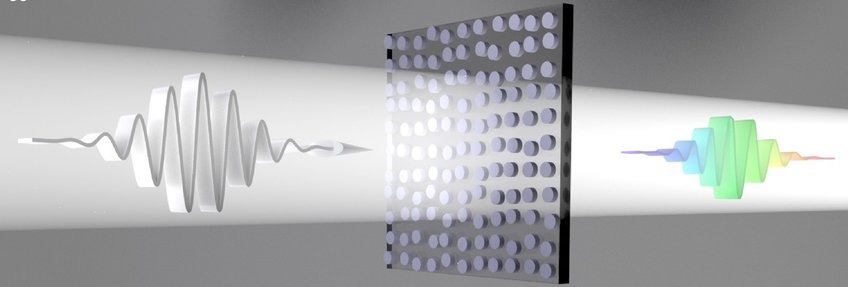
Nanophotonics in Complex Media
Light scattering and transport through nanostructured materials and interfaces are crucial in various fields of research and technology, as well as in everyday life. Understanding the complex interactions of electromagnetic waves with nanostructures is challenging but essential for advancing technologies and products in multiple sectors, and for answering fundamental questions across scientific disciplines. By studying light scattering in ordered and disordered media, we can develop applications ranging from integrated optics and diagnostics to consumer products and lighting solutions.
Nature offers inspiration for greener and more sustainable approaches through the patterns of scattering, interference, and diffraction. Depending on the nanostructure's composition, we use different modelling and simulation techniques to understand and predict optical responses, and we develop optical setups to characterise complex systems experimentally. Here, we address the following four key research questions explored in our department:
1. Light Transport in Complex Multilayered Systems
Multilayered films with varying optical properties and thicknesses offer a playground for unique light transport phenomena, such as forbidden wavelengths or artificial optical transparency. These nanostructures, prevalent in nature, can be addressed using the transfer matrix method.
Tools:
Transfer Matrix Method (PyLlama)
2. Light Transport in Complex Media with "Independent Scatterers"
In media where individual scatterers are optically distanced, one can assume negligible interaction between these "independent scatterers." This assumption simplifies the governing equations for light radiative transfer, and for sufficiently thick samples, the Monte Carlo method can be used to address such problems.
Tools:
3. Light Scattering in Complex Materials and on Surfaces with "Dependent Scatterers"
Resonant structures contain strongly interacting scatterers, making them computationally very expensive. When these scatterers are geometrically non-overlapping, coupled-dipole theory (for smaller nanoparticles) and the Transition Matrix (T-matrix) Method can be used to address their coupling and quantify light scattering. Photonic crystals, photonic metamaterials, metasurfaces, and photonic glass (disordered photonic crystals), whose periodicity and parameters offer control over polarisation, reflection, or transmission, are examples of systems with dependent scatterers.
For periodic structures, certain simplifications lead to analytical equations enabling a better understanding of the underlying physics of resonant structures.
Tools:
Mie theory, Coupled-Dipole Theory, Transition Matrix Method (Treams, Celes), Reticolo
4. Full-Wave Simulations
Although full-wave simulations can, in principle, address all of the above scenarios, they involve a huge computational burden that is not suitable for larger disordered systems.
Tools:
Full-wave simulations (COMSOL Multiphysics®, Ansys Lumerical)
5. Light Transport and Management in Photosynthetic Organisms
Light management is a crucial mechanism for biological systems relying on energy from photosynthesis. Nature has developed a plethora of mechanisms to optimise the internal light field of such organisms, including the growth of fascinating micro- and nanostructures. Shedding light on the connection between structure and optical function requires disentangling the interplay of biological mechanisms and optical processes like scattering and absorption in complex living systems.
Tools:
Full-wave simulations, Monte Carlo (Theory)
Transmission and fluorescence spectroscopy, holotomography, microsensing (Experimental)
