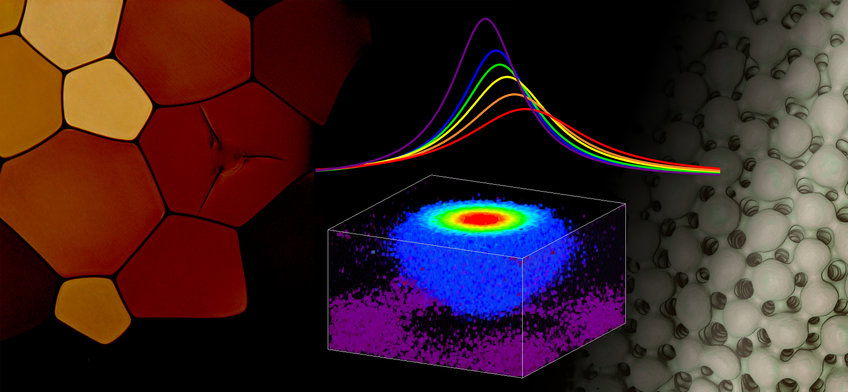
Micromechanics of Biological and Bio-inspired Materials
The way a matter undergoes mechanical deformations and accommodates strains is central to its performance and sets the border between its function and failure. Thus, studying these deformations, whether generated internally or applied externally, reversible or irreversible, is essential for understanding the materials' behavior and setting the way for designing new materials and devices with encoded functions. Our team, fascinated by the physical and material principle of mechanical behavior, tries to uncover the evolution of deformations, from their i) formation and development to ii) storage and accumulation and iii) relaxation and functions. Our group primarily investigates biological models representing complex materials in which interfaces, anisotropies, heterogeneities, and structural hierarchy are embodied, and their deformations can be direction, location, and condition-specific and vary across length scales (molecules to tissue). We transfer the achieved design concepts and materials' information to proof-of-concept fabrication and synthesis using additive and micro-mold manufacturing, polymerization, and gel-mediated mineralization.
By leveraging our expertise in mechanical engineering, materials science, physics, and biology, our studies are centered on the following areas:
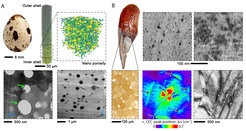
- Strain development in complex materials: Our team's core aim is to provide a general framework for a quantitative understanding of the formation and development of mechanical deformations in architectured materials that embody interfaces, anisotropies, gradients, and heterogeneities. Our investigations include i) studying the presence and distribution of internally generated stresses and their interaction with applied forces, ii) investigating the evolution of elastic deformation fields, and iii) characterizing the development of early-stage inelastic deformations (plasticity, quasi-plasticity, and brittle fracture), in particular localized quasi-plasticity, deformation twinning, nano-\microcracking, wear, and abrasion.
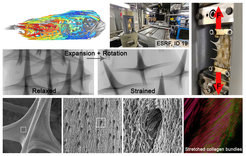
- Functional interfaces and encoded functions at interfaces: Biological materials are formed through a bottom-up approach, permitting the development of hierarchical structures with complex architectures and superior properties compared to their artificial counterparts. Yet, this development comes with its inevitable consequences, namely interfacing materials with incompatible properties. We investigate how deformation and motion at interfaces with mismatched properties can promote material functionality while sustaining structural integrity.

- Materials multifunctionality: Biological materials greatly offer a broad spectrum of fascinating approaches for assembling their building blocks, so materials' architecture and, accordingly, their properties can be locally altered and modified. This capability rewards biological materials with striking multifunctionality paradigms in which a combination of dissimilar characteristics such as adaptivity, mechanical integrity, light manipulation, sensing and actuation, fluid transportation, and anti-biofouling can be integrated into materials levels. We try to uncover the physical principle of multifunctionality by which unlike functions (such as optical and mechanical functions) can be incorporated into materials' microarchitecture.

- Methodological development for elastic deformation analysis (Materials @ Work): Characterizing the mechanical behavior of biological materials requires correlative and multi-scale approaches capable of resolving hierarchical and spatiotemporal complexities. This can be even more challenging when elastic deformations are targeted, so the characterizations should be performed under mechanical loads. With extensive experience in studying micromechanics of biological materials, our team has developed and patented the methods that allow visualizing and quantifying these deformations at different length scales and under controlled stimuli conditions. Examples include our in-operando nanoindentation Wide Angle X-ray Scattering (WAXS) and indentation Raman methods (Figure 4) to map the development of elastic strain fields and study their interaction with residual strains
- Contact and materials loss: This project, led by Dr. Maryam Tadayon, focuses on investigating the mechanical and tribological properties of biological materials, particularly exploring how micro-architectural features and compositional heterogeneity alter their wear resistance. By employing advanced microscopy and nanomechanical techniques, we analyze the relationship between structure, stress distribution, and mechanical performance in natural systems such as cuticular materials. Our interdisciplinary approach integrates experimental studies and finite element modeling to uncover the fundamental principles behind structural adaptations in nature. This knowledge guides the development of innovative bioinspired materials with superior durability, functional gradients, and potential applications in engineering fields, including coatings, robotics, and sustainable materials design.
Outreach
Learning from nature rewards us with attaining artful design principles that can be implemented to craft novel functional materials. Our team uses these design principles not only for designing proof-of-concept models for fabricating bio-inspired materials and devices but also to arrange courses for students in different fields, from architectural designs to biomaterials and physical sciences. Moreover, our team actively participates in outreach, educational workshops, and programs such as Open Science, where we engage with public audiences and present our work to a broader audience with no/least knowledge background.
Our team @ Max Planck Queensland Center (MPQC)